Unleashing the Potential of Antimatter: A Sustainable Energy Solution
Antimatter, the mirror counterpart of matter, is composed of particles with opposite charges, such as positrons and antiprotons. When matter and antimatter collide, they annihilate each other, converting their mass into pure energy according to Einstein’s equation, $$E = mc^2$$. This article explores how antimatter could become a sustainable energy solution, along with the challenges and advancements in this emerging field.
What Is Antimatter?
Antimatter Basics
Antimatter consists of antiparticles that have the same mass as their corresponding particles but opposite charges. When antimatter encounters matter, they annihilate each other, releasing energy in the form of gamma rays. This annihilation is one of the most energy-dense processes known to science.
Discovery of Antimatter
The existence of antimatter was theoretically predicted by physicist Paul Dirac in 1928, leading to the discovery of the positron in 1932. Today, antimatter is routinely produced and studied in particle accelerators like CERN’s Large Hadron Collider, albeit in minuscule quantities.
Energy Potential of Antimatter
Energy Density of Antimatter
Antimatter boasts an enormous energy density. The annihilation of just 1 gram of antimatter could release energy equivalent to 43 kilotons of TNT—about three times the energy released by the Hiroshima bomb. This process is vastly more energy-dense than conventional nuclear fission or fusion; for instance, 1 gram of antimatter could generate as much energy as thousands of tons of chemical fuel.
Applications in Energy
Antimatter’s high energy density makes it an ideal candidate for space propulsion, where fuel efficiency is crucial. Antimatter-powered spacecraft could achieve higher speeds, drastically reducing travel time to distant planets or even other star systems. Furthermore, while still theoretical, antimatter reactors could provide an almost limitless supply of clean energy without producing radioactive waste.
Challenges in Using Antimatter as an Energy Source
Antimatter Production
Producing antimatter is currently extremely expensive and energy-intensive. The estimated cost to produce just 1 gram of antimatter is around $62.5 trillion due to the massive energy requirements involved. Moreover, modern particle accelerators can only produce nanograms of antimatter per year, which is far below what would be needed for practical applications.
Storage Issues
Since antimatter annihilates upon contact with matter, it must be stored in a vacuum and suspended using magnetic fields. Developing reliable and safe containment methods for large-scale storage remains a significant technological hurdle.
Safety Concerns
The potential for destructive energy release poses safety concerns. Improper handling or containment failure could lead to catastrophic explosions due to the immense energy released during annihilation.
Technological Advancements and Research
Advancements in Antimatter Production
Researchers are exploring ways to enhance the efficiency of antimatter production through advanced particle accelerators and investigating natural sources of antimatter generated by cosmic rays or within Earth’s atmosphere. Emerging research suggests that high-powered lasers may also facilitate more efficient antimatter production by stimulating high-energy collisions.
Antimatter Storage Solutions
Innovations such as Penning traps and magnetic bottles have been developed to store tiny quantities of antimatter by keeping it suspended in magnetic fields. These technologies could eventually lead to more stable and scalable storage solutions.
Fusion with Other Technologies
Combining antimatter with other emerging energy technologies, such as nuclear fusion, could create hybrid reactors that maximize energy output while addressing current production and storage challenges.
Is Antimatter a Sustainable Energy Solution?
Long-Term Sustainability
If production can be scaled efficiently, antimatter could provide clean, carbon-free energy. Unlike fossil fuels, its generation would not emit greenhouse gases or pollutants. Researchers are also investigating whether renewable sources like solar or wind can power the energy-intensive processes involved in its creation.
Economic and Technological Feasibility
Despite its immense potential, current costs are prohibitive. Major technological breakthroughs in production, storage, and safety are necessary before antimatter can become a viable part of the global energy mix. Given these challenges, antimatter may initially find applications in specialized areas like space travel before broader uses are realized.
Future of Antimatter in Energy and Beyond
Potential for Space Exploration
Antimatter propulsion could revolutionize space travel by making interstellar exploration feasible within human lifetimes. Antimatter engines would be lightweight and powerful enough to achieve a fraction of light speed.
Antimatter in Medicine
Currently used in Positron Emission Tomography (PET) scans for detecting diseases like cancer, advancements in antimatter technology could lead to new possibilities in medical treatments and diagnostics.
Frequently Asked Questions (FAQs)
1. What is antimatter, and how does it work as an energy source?
Antimatter consists of particles with opposite charges compared to normal matter. When they collide, they annihilate each other, converting their mass into pure energy.
2. Why is antimatter considered a potential energy solution?
It offers the highest energy density among known substances; even small amounts can produce massive amounts of energy.
3. What are the biggest challenges to using antimatter for energy?
The primary challenges include high production costs and energy requirements, along with difficulties in safe storage due to its annihilation properties.
4. Is antimatter a renewable energy source?
Currently inefficient and energy-intensive to produce, research is ongoing to improve methods and explore renewable sources for its creation.
5. How might antimatter be used in space travel?
Its high energy density makes it ideal for space travel where efficiency is critical; it could significantly reduce travel times to distant destinations.
Conclusion
The incredible energy potential of antimatter presents both opportunities and challenges for sustainable energy solutions. Continued research is essential to overcome technological and economic barriers related to its production, storage, and safety. While still largely theoretical, antimatter has the potential to transform both energy generation and space exploration in the future.
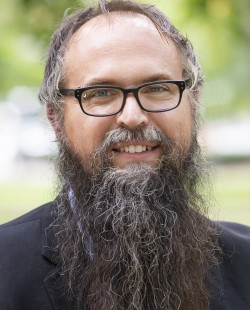
Kyle Whyte is a notable scholar and professor at the University of Michigan, holding positions such as the George Willis Pack Professor in the School for Environment and Sustainability and Professor of Philosophy. Specializing in environmental justice, his work critically examines climate policy and Indigenous peoples’ ethics, emphasizing the nexus between cooperative scientific endeavors and Indigenous justice. As an enrolled Citizen Potawatomi Nation member, he brings a vital perspective to his roles as a U.S. Science Envoy and member of the White House Environmental Justice Advisory Council. His influential research is supported by various prestigious organizations including the National Science Foundation, and disseminated through publications in high-impact journals. Kyle actively contributes to global Indigenous research methodologies and education, with affiliations to numerous institutes and societies dedicated to traditional knowledge and sustainability. Recognized for his academic and community engagement, Kyle has earned multiple awards and served in various visiting professorships. His efforts extend to leadership positions on boards and committees focused on environmental justice nationwide.